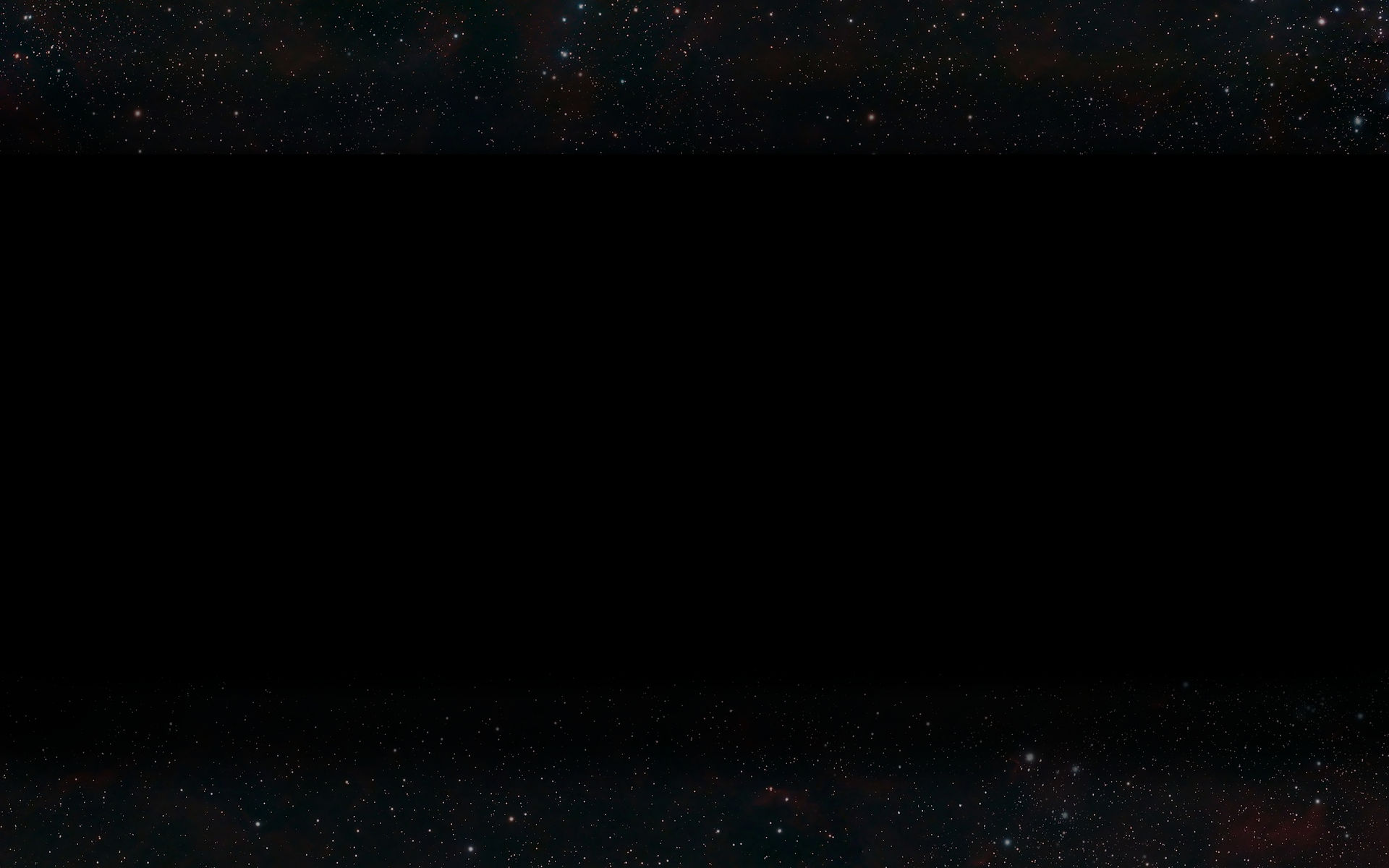
2nd Generation (gen2) Systems:
Evolution to Higher Temperatures and/or Powers

A Gen2 reactor is one that, based on our experience, would require >4 years and >$500M dollars to produce a flight system. It is extremely hard to sustain funding for a program that size unless everything goes exactly as planned, which has never happened in the history of reactor development. Fortunately, the lessons learned during a Gen1 flight program can give us the experience to confidently choose the technologies and predict the resources needed to successfully complete the initially more-risky Gen2.
The key attribute of a Gen2 design is that reactor dynamics remain sufficiently close to Gen1, so that a nuclear-powered ground test is in not required; this is the game-changing advantage that the Kilopower approach has over other concepts, especially moderated reactors (because the physics change every time the size or moderator ratio changes).


5 to 100 kWe HEU High-Temperature Small-Radiator Concept
High-temperature Gen2 concepts (from 1300 to 1500 K reactor outlet) use the same compact, fast-spectrum physics as KRUSTY, with the added risk of higher-temperature materials. These Gen2 systems can have radiator sizes up to 10 times smaller than Gen1, which opens the door to numerous advanced NEP applications.
The primary benefit of higher reactor temperature is the ability to increase the cold-end temperature of the Carnot cycle, which allows a substantially smaller radiator. Other than the radiator, the size and configuration of Gen2 HEU power systems would look very similar to the Gen1 HEU systems.
The challenge of high temperature lies in 3 areas. 1) A change to a higher temperature fuel: this challenge is not based in technology development, rather the challenge is creating a new fuel line and other required infrastructure within acceptable cost and schedule. 2) A change to a refractory (very high temperature) structural material: this challenge requires development in manufacturing and joining processes. 3) An advancement in power conversion technology: this challenge requires development of either high temperature alternators or alternator systems with integrated cooling loops.
Each of the required technology areas can be researched in parallel as Gen1 systems are developed and flown. SpaceNukes is currently investing in many of these areas, but our focus remains on flying a Gen1 system to build the expertise and infrastructure needed to succeed on Gen2 systems and beyond


100 to 300 kWe “Low-Temperature” HALEU Surface Reactor

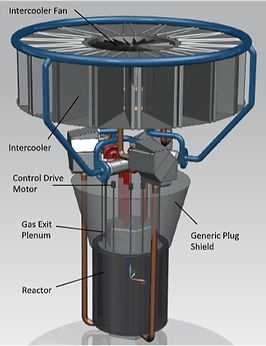
Figure 1 - 250 kWe HALEU Surface Reactor with Top Shield and Intercooler Fan for Mars
Higher-power Gen2 concepts (>100 kWe) can still use the same fast-spectrum physics as KRUSTY and “established” materials , but lack the technology, infrastructure, and capabilities to be completed on a realistic Gen1 cost and schedule.
The 250-kWe concept shown in Figure 1 uses approximately 2 metric tons of uranium oxide fuel in a stainless-steel monolithic block. The relatively large mass of fuel allows for a reactor lifetime of 20+ years without refueling. This concept uses Brayton technology for power conversion and an intercooler designed to reject heat to the sparse Martian atmosphere. Lunar concepts would have to develop a very large radiator system or pump coolant “off-board” to a heat sink (ideally to an application that could utilize the waste heat, or perhaps just laying piping across the regolith).
The concept in Figure 1 transports reactor power by flowing gas through dispersed monolith holes, although it might be better to use heat pipes to transport heat to a gas heat exchanger. It is impossible to predict the most-likely-to-succeed heat transport approach until a Gen1 system is developed and flown (e.g. lessons learned about in-space reactor instrumentation and control) and Brayton technology is adequately developed (e.g. lessons learned about dynamic and reliability characteristics). It is anticipated that the monolith would be printed as one solid block. The block would be about 50 cm in diameter and about 1 meter high.​
This power system is in the conceptual design phase and would require more engineering before a cost and schedule estimate could be developed. Given that the reactor is a fast neutron reactor with a monolithic fuel block, the reactor would self-regulate in the same fashion as the Kilopower reactor concepts. The practical evolutionary limit of this technology would be in the range of a few MWe.
